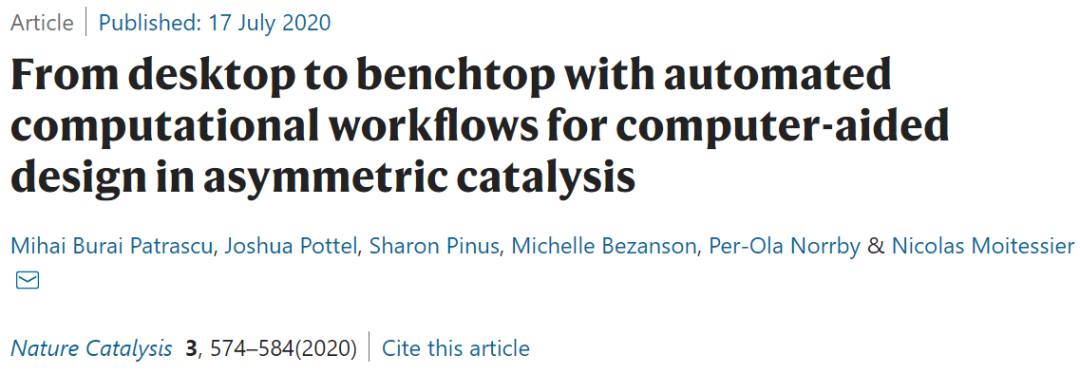
Background
To design novel organic synthesis methods for obtaining new compounds, chemists typically utilize a wide array of organic chemistry tools. Although computational chemistry has been used to help explain certain phenomena and propose new mechanisms, it is less accessible to experimental chemists compared to techniques like NMR, MS, and mass spectrometry due to the need for programming knowledge. In the field of asymmetric synthesis, which is a powerful method for producing large quantities of high-purity chiral molecules, discovering organic or transition metal catalysts using only traditional experimental techniques is highly challenging and tedious. There is an urgent need for a computational tool that is simple, efficient, accurate, and practical.
Preliminary Considerations and Interface Overview
For organic chemists, a tool must address accessibility without sacrificing accuracy. This challenge can be addressed through the interaction between chemists and technology, such as specifying desired compound properties. Ideally, a platform should seamlessly execute all three processes of catalyst discovery (preparing a library of potential catalyst molecules, evaluating catalytic activity, and assessing enantioselectivity) without manual intervention. The authors have extended their drug discovery platform, FORECASTER, to create a new platform called Virtual Chemist. The user interface (UI) of this platform includes a 2D structure drawer for inputting catalyst and substrate structures, and a 3D graphical interface for visualizing the computed transition state (TS) structures. The UI also summarizes the resulting data (e.g., potential energy and enantioselectivity of the TS structures). The entire workflow has been modularized to be easily used by researchers.
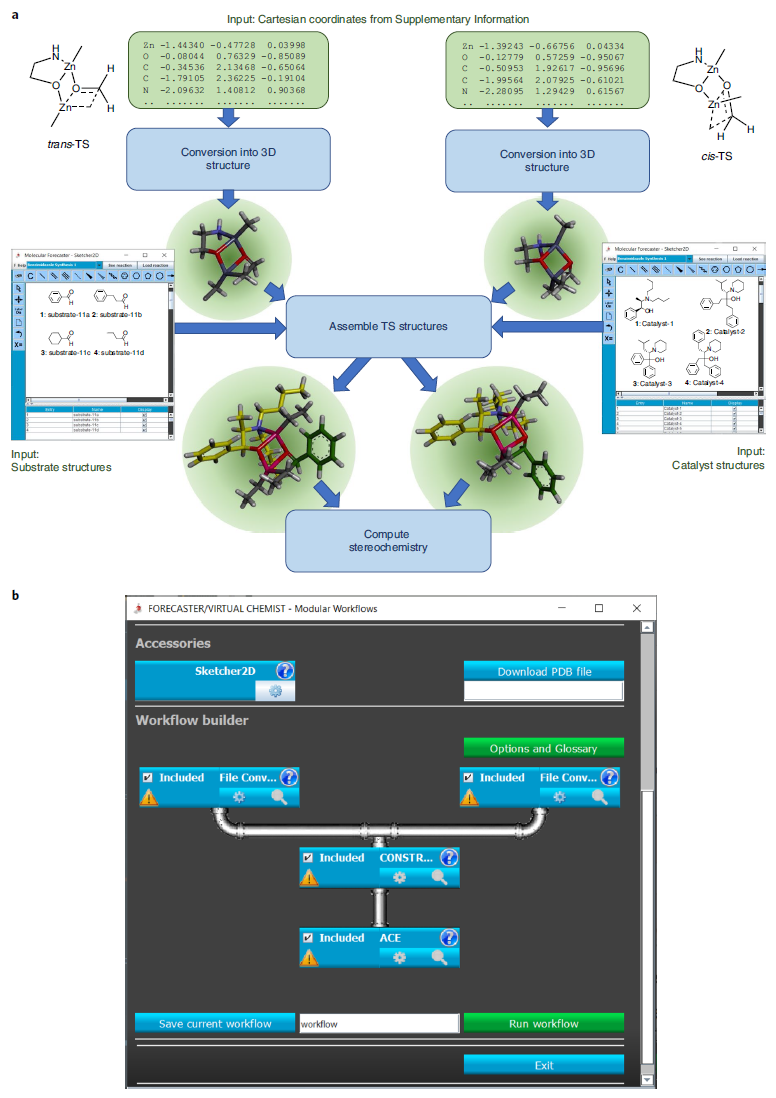
Figure 1. Catalyst Screening Process for the Addition of Diethylzinc to Aldehydes
Image Source:Nature Catal.
Four Application Scenarios
- One-on-One Design
Chemists may wish to test one catalyst at a time to determine the most promising one. In this case, each catalyst can be drawn using the 2D structure drawer, and the transition state (TS) template can be directly used or constructed from literature data. The authors tested over 350 reactions across 7 classes of reactions and compared the results with random predictions to evaluate the method’s accuracy. They found that the platform’s overall average error ranged from 0.94 to 0.97 kcal/mol, with 1 kcal/mol being the gold standard for quantifying and catalyzing reactions. Additionally, the platform was able to distinguish between poor asymmetric catalysts (0% e.e., ΔΔG‡ ~0 kcal/mol) and good ones (90% e.e., ΔΔG‡ ~1.4 kcal/mol), as well as excellent asymmetric catalysts (99% e.e., ΔΔG‡ ~2.8 kcal/mol) from good ones. The data indicate that the platform can be used to evaluate asymmetric catalysts through interaction with chemists and facilitate larger-scale virtual screening.
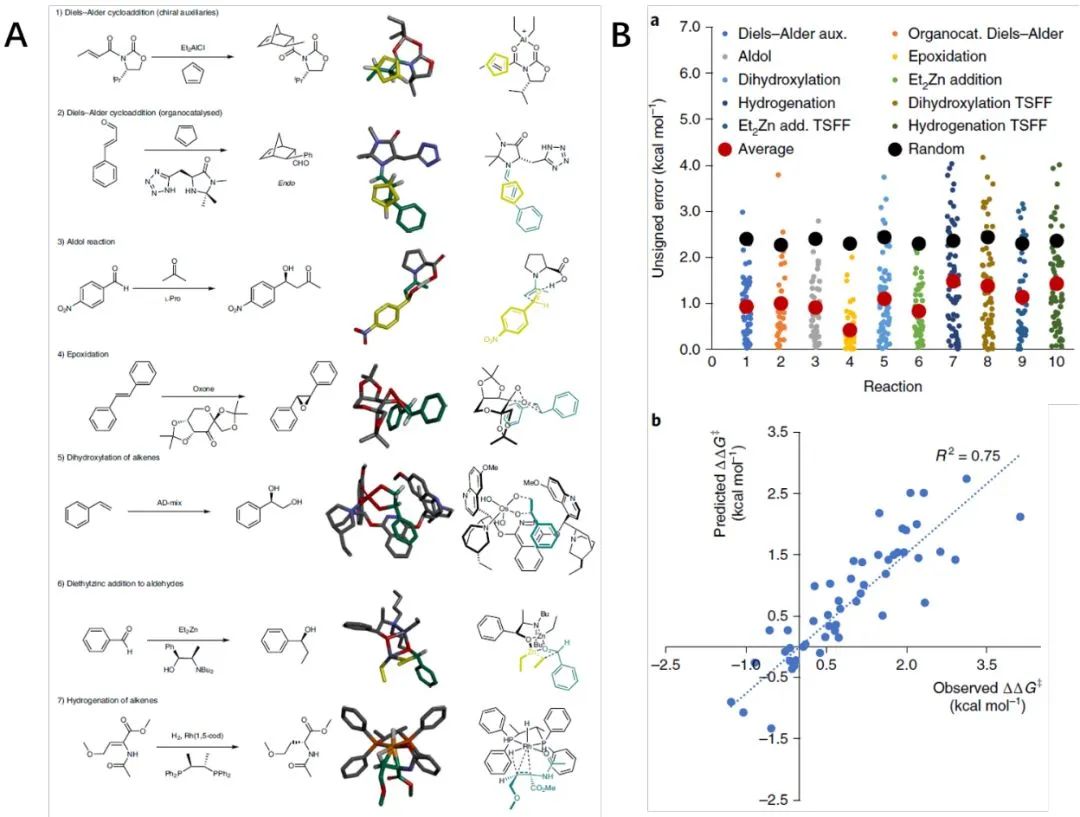
Figure 2. A: 2D and 3D Representations of the TS States for Seven Major Reaction Classes; B: Accuracy of One-on-One Design
Image Source:Nature Catal.
- Screening Chiral Molecular Libraries
Chemists may be searching for new chemical series as catalysts for known reactions, such as the Shi epoxidation and organocatalyzed Diels-Alder reactions. The authors assembled a library of approximately 140,000 chiral amines from the ZINC database for the Diels-Alder reaction. They screened the molecules of interest by calculating molecular descriptors (MW < 500, no charge, excluding secondary amines, aldehydes, and other reactive groups), then removed molecules too similar to any known catalysts and selected the most diverse molecules. The program DIVERSE ensured no duplicates were left, resulting in 1,307 candidate catalysts for the next round of screening. The evaluation of these amines was conducted in two steps: filtering for reactivity to yield 798 molecules, and using ACE to calculate the stereoselectivity of each molecule’s TS. Six catalysts were added to the library to assess ACE’s accuracy in recovering these structures. The same process was used to search for Shi epoxidation catalysts starting from chiral ketones, ultimately adding 18 known stereoselective catalysts to the library. Figure 3 shows that most known stereoselective catalysts ranked highly (Figure 3c, AUROC: 0.79 for Shi epoxidation, 0.92 for Diels-Alder), indicating that the platform can screen a variety of chemicals and discover asymmetric catalysts for new chemical series.
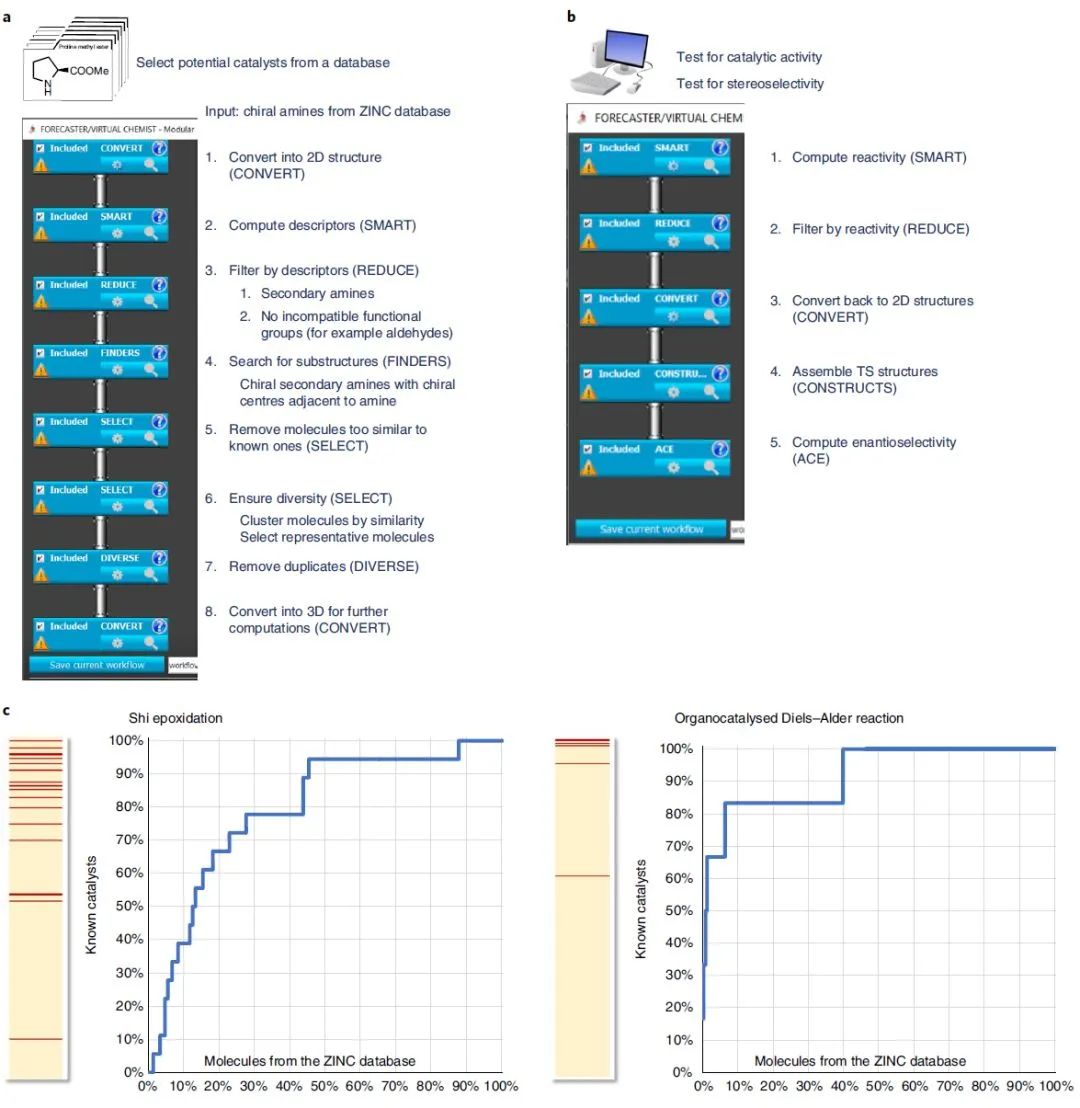
Figure 3. Accuracy of Screening for Novel Asymmetric Catalysts
Image Source:Nature Catal.
- Lead Compound Optimization
Chemists may have a lead compound that needs structural optimization to enhance selectivity. In this scenario, chiral pyrrolidine derivatives were synthesized and tested as organocatalysts for the Diels-Alder cycloaddition reaction. First, imines were synthesized, and then they were reacted with chiral dipolar nucleophiles to generate three potential diastereomers. These pyrrolidines were evaluated in both endo-Diels-Alder and exo-Diels-Alder cycloadditions, significantly reducing experimental workload. The data showed that the average error in optimizing the lead compound was as low as 0.33 kcal/mol. This project could be completed within a few days on a standard PC and is scalable to hundreds of analogs.
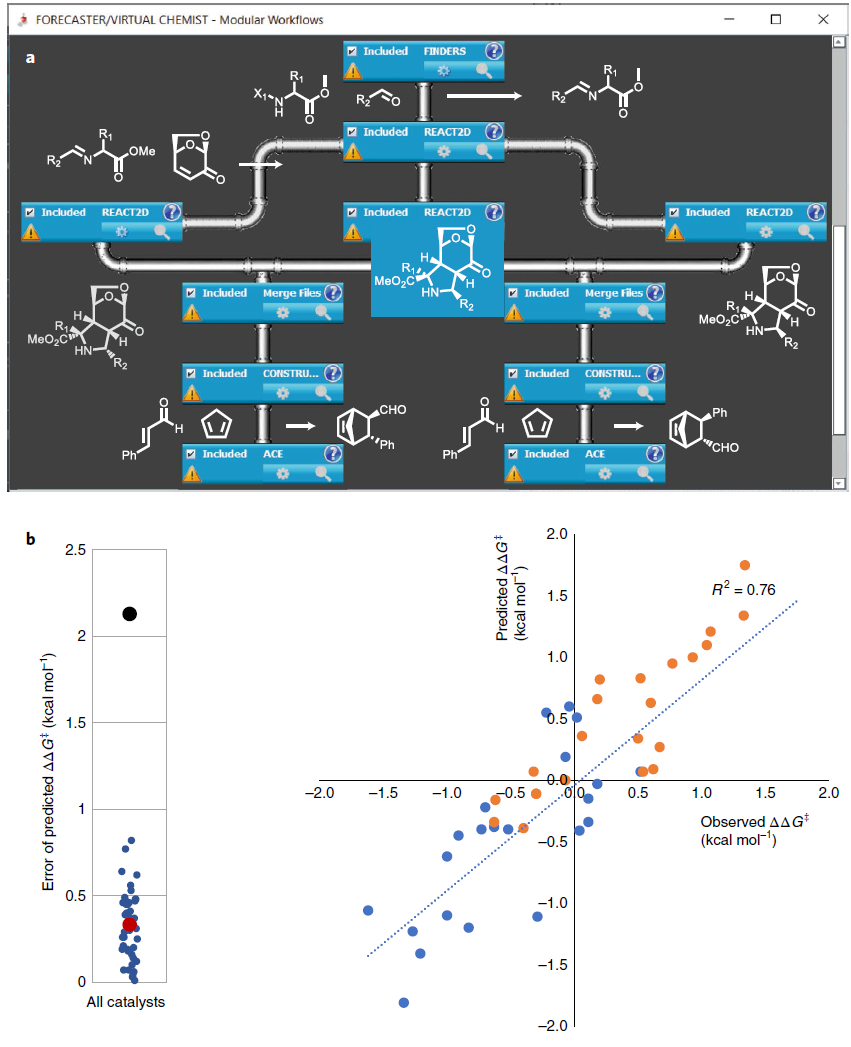
Figure 4. Optimization of Asymmetric Organocatalysts for the DA Cycloaddition Reaction
Image Source:Nature Catal.
- Evaluating Catalyst Substrate Scope
Chemists can use the platform to evaluate the potential substrate scope of a given catalyst. In this example, the commercially available catalyst (DHQD)2PHAL, used for asymmetric dihydroxylation reactions, was chosen for simulation. The simulation results indicated that this catalyst exhibits very high enantioselectivity (≥97% e.e.) for approximately 25% of the substrates, while it shows poor selectivity (≤40% e.e.) under about 20% of the conditions. These findings align well with experimental data. However, compared to other reactions, the reproducibility of dihydroxylation results is lower, likely due to the larger and more flexible nature of the catalyst molecules used in this reaction, which highlights a limitation of the method. Fully exploring the conformational space of such systems may require more time and computational resources. Each calculation was run five times, with predicted enantioselectivity averaging between 67% and 76% e.e., while the experimental value was 73.6% e.e., showing good correlation with experimental data (r²: 0.51~0.64).
Summary
The Virtual Chemist platform aims to assist experimental chemists in leveraging computational chemistry tools for asymmetric catalysis projects. The platform features a user-friendly workflow that can be highly customized and has demonstrated accuracy in four real-world scenarios. While MM-based calculations are limited by current force field constraints and the potential for simulations to get trapped in local energy minima, the platform generally identifies good asymmetric catalysts with reasonable accuracy. Chemists can test their ideas within a few hours.
References:Mihai Burai Patrascu, Joshua Potte, Sharon Pinus, Michelle Bezanson, Per-Ola Norrby and Nicolas Moitessier, From Desktop to Benchtop with Automated Computational Workflows for Computer-aided Design in Asymmetric Catalysis. Nature Catalysis, 2020, 3, 574–584. DOI: 10.1038/s41929-020-0468-3.